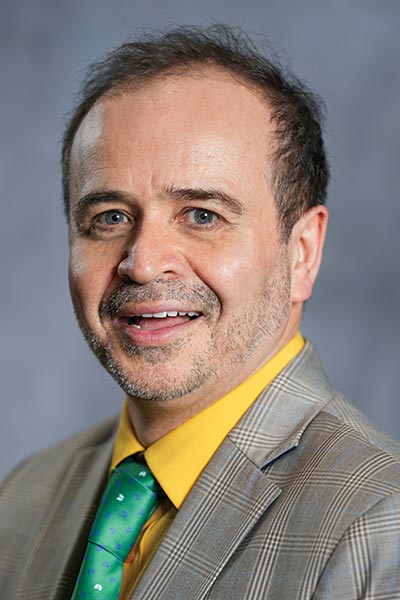
COPD affects approximately 29 million people and is the fourth leading cause of mortality in the United States. Despite its public health burden, treatment options for COPD remain limited, largely due to several challenges: an incomplete understanding of the disease’s underlying mechanisms, difficulties in translating animal model findings into humans, and a lack of sufficient investment in research and drug development. One promising yet understudied area for therapeutic intervention is mucus plugging—a unique form of airway mucus pathology that could offer a new target for treatment.
In individuals with COPD, pathologic changes in the mucociliary system lead to hyperconcentrated and stickier mucus and reduced ability to clear it from the airways. This characteristic hyperconcentrated mucus is primarily due to imbalances in fluid transport in the airway epithelium and heightened levels of mucins—polymers that give mucus its gel-like consistency. Mucin concentrations in sputum correlate closely with COPD severity and chronic bronchitis and can predict future exacerbations in individuals who are symptomatic and exposed to tobacco, even before airflow obstruction becomes apparent.1 Additionally, cigarette smoke damages cilia and disrupts the function of the cystic fibrosis transmembrane receptor, further impairing mucus clearance. The result is mucus accumulation, plaque formation, and the development of mucus plugs (Figure 1). The occlusion of small airways by mucous content is a key contributor to the obstructive pathophysiology of COPD.
However, translating these mechanistic insights to clinical trials has been challenging, primarily due to the difficulty in accurately measuring mucus pathology. For example, quantifying mucins in sputum requires intricate protocols for sample collection, preservation, transport, and processing, which limits its practical use in clinical practices. Similarly, relying on questionnaires to assess mucus-related symptoms, such as cough and phlegm, is hindered by recall bias and inconsistencies in reproducibility. These challenges have made it difficult to move forward with mucus pathology as a therapeutic target for COPD.
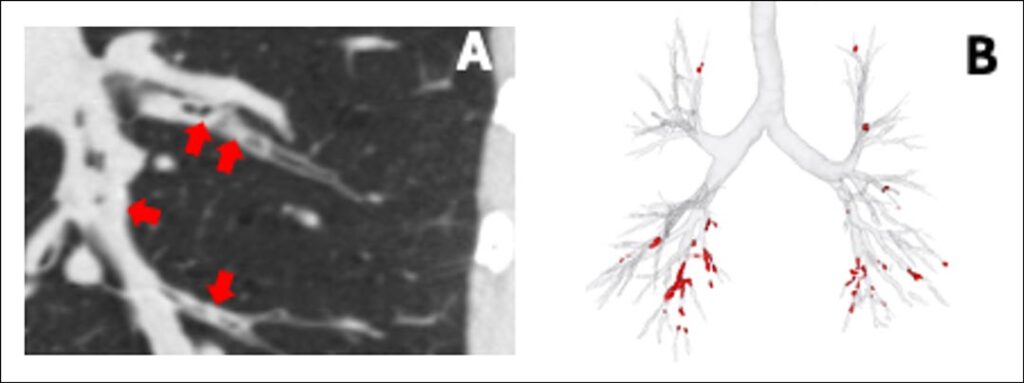
In recent years, chest CT scanning has become an invaluable tool for identifying and quantifying mucus plugs in medium-to-large-sized airways (~2-10-mm lumen diameter). In the COPDGene, Evaluation of COPD Longitudinally to Identify Predictive Surrogate Endpoints (ECLIPSE), and Subpopulations and Intermediate Outcomes in COPD Study (SPIROMICS) cohorts, it has been found that the prevalence of CT scan-detected mucus plugs varies: 41%, 46%, and 67%, respectively, underscoring the relevance of this pathology.2–4 CT scan-detected mucus plugs block the airways, increasing airflow resistance and decreasing lung function. In the COPDGene and SPIROMICS cohorts, patients with mucus plugs exhibit lower FEV1 and more severe disease than those with cleared airways.4,5
Mucus plugs vary in appearance: Some are short (“stubby,” <12 mm in length), while others are long (“stringy,” ≥12 mm in length). The latter tend to contribute more to the total mucus volume observed on CT scans.6 Mucus plugs mainly localize in airways 2 to 4 mm in lumen diameter.6 The formation and disappearance of mucus plugs are dynamic; some may resolve quickly, while others can persist over the years. Follow-up data show that 67% of patients with COPD still have mucus plugs after one year, and 73% still have them after five years.4,5 Interestingly, mucus plugs in the lower lobes tend to be more persistent than in other regions.5 It is thought that the composition of mucus plugs—comprising dead cells, mucins, fibrin, extracellular DNA, and microbes—and their crosstalk with airway epithelial cells might play a role in the persistence. Regardless of the underlying mechanism, patients with persistent mucus plugs appear to have a faster decline in FEV1, substantiating their causal role in the obstructive pathophysiology of COPD. A further functional consequence of airway-occluding mucus plugs is a decrease in oxygen saturation. Notably, this drop in oxygen saturation is more pronounced in patients without emphysema, likely due to the ventilation/perfusion mismatch caused by the lung parenchyma destruction.4
Despite the well-established link between cough, sputum production, and mucociliary dysfunction, up to 36% of people with COPD who have mucus plugs on CT scan report no cough or phlegm. These “silent mucus plugs” might be particularly concerning, as they have been linked to reduced FEV1 and oxygen saturation, lower exercise capacity (as measured by a 6-minute walk distance test), and poorer quality of life (as measured by the St. George’s Respiratory Questionnaire), supporting mucus plugs as a unique form of pathology.7
Patients with mucus plugs are at higher risk for adverse outcomes, including exacerbations, all-cause mortality, and respiratory deaths.2–4,7,8 Recent studies in the COPDGene and ECLIPSE cohorts have shown that patients with mucus plugs experience more frequent moderate to severe exacerbations and severe exacerbations (ie, those requiring hospitalizations). Over time, these patients have a 7% to 15% higher risk of moderate to severe episodes and a 5% to 37% higher risk of episodes requiring hospitalization compared with those without mucus plugs.3 The association holds across various patient groups—women and men, patients with a BMI below and ≥30, individuals who formerly smoked, those with mild to moderate and severe or very severe COPD, and individuals with and without a history of exacerbations.
Furthermore, a separate study found that mucus plugs are linked to higher all-cause mortality in patients with COPD. Specifically, the mortality risk increased by up to 26% in patients with mucus plugs affecting three or more lung segments.2 A subsequent study of the same cohort revealed that mucus plugs also contribute to respiratory deaths, with a 13% and 36% increased risk when one to two and three or more lung segments were involved with mucus plugs.8 The potential mechanisms underlying these associations may include plugs being a nidus of infection and inflammation, potentiating microbial growth. Moreover, these airway-occluding mucus plugs can worsen ventilation/perfusion mismatch, which may lead to respiratory failure.
Current CT scan-based studies rely on time-consuming visual methods to identify and quantify mucus plugs, including counting affected lung segments or individual plugs. However, recent advances in artificial intelligence (AI)-based approaches seem promising in overcoming these limitations, improving the efficiency and accuracy of mucus plug detection (Figure 1). Regardless of the methodology used, airway mucus plugs on CT scans are linked to functional measures of COPD and clinically relevant outcomes, supporting them as a potential therapeutic target.
Several therapies have the potential to treat mucus pathology, including biologics and muco-active drugs and more broadly accessible COPD treatments, including nebulized saline and positive expiratory pressure devices (flutter valves). These therapies can reduce the burden of mucus plugs in the airways, but they have yet to undergo rigorous testing in COPD-specific clinical trials. Encouraging results from a trial in moderate to severe asthma suggest that biologics, such as those blocking the thymic stromal lymphopoietin receptor—which activates interleukine-13 and interleukine-5 signaling—can decrease mucus plugs and improve lung function.9 Additionally, muco-active drugs have shown efficacy in reducing airway mucus plugs in animal models.10 Regardless of the treatment approach, removing accumulated airway mucus could have wide-ranging benefits. It may lower airway microbial load, increase expiratory airflow, improve the function of the ciliated epithelial cells, and potentially promote a more uniform airway distribution of inhaled medications, thereby improving overall treatment effectiveness.
Designing clinical studies to test interventions targeting mucus plugs presents several challenges. First, mucus plug scores are generally not utilized in routine clinical practice, meaning that this information is rarely available in medical records, making it challenging to identify potential patients for clinical trials. Second, there is a clear need for a standardized approach to define, quantify, and report this imaging biomarker. Third, identifying and quantifying mucus plugs requires training, which can be a barrier for widespread implementation. Lastly, while AI-based algorithms have shown promise in detecting mucus plugs, the widespread adoption of this technology is limited, hindering their potential to streamline and improve the detection process in clinical settings.
While substantial progress has been made, there is still much to uncover about the nature of mucus pathology. The content of mucus plugs is assumed to be just “mucus,” yet a deep characterization of their components is still lacking. It remains unclear whether CT scan-detected mucus plugs truly reflect small airways pathology and why some persist over time. Emerging fields like omics science—proteomics, metabolomics—hold promise to discover the molecular pathways that drive mucus pathology. Most studies of mucus plugs have focused on older individuals, individuals with chronic airway diseases, and those exposed to tobacco; however, we have little understanding of how mucus pathology manifests in the broader community-dwelling population. Additionally, the potential role of environmental and lifestyle factors—such as indoor and outdoor air pollution, cannabis use, vaping, and physical activity—on mucus plug formation remains largely unexplored. Addressing these gaps could lead to new insights into how mucus plugs develop and how we can better treat them in diverse patient groups.
Targeting mucus pathology could fulfill an unmet therapeutic need in managing patients with COPD, providing a novel approach to addressing the underlying mechanisms contributing to airway obstruction. By focusing on clearing sticky, mucus-laden airways, we could modify disease course and improve patient outcomes.
This article was originally published in the Spring 2025 issue of CHEST Physician.
References
1. Kesimer M, Ford AA, Ceppe A, et al. Airway mucin concentration as a marker of chronic bronchitis. N Engl J Med. 2017;377(10):911-922.
2. Diaz AA, Orejas JL, Grumley S, et al. Airway-occluding mucus plugs and mortality in patients with chronic obstructive pulmonary disease. JAMA. 2023;329(21):1832-1839.
3. Wan E, Yen A, Elalami R, et al. Airway mucus plugs on chest computed tomography are associated with exacerbations in COPD. Am J Respir Crit Care Med. Preprint. Posted online October 29, 2024.
4. Dunican EM, Elicker BM, Henry T, et al. Mucus plugs and emphysema in the pathophysiology of airflow obstruction and hypoxemia in smokers. Am J Respir Crit Care Med. 2021;203(8):957-968.
5. Okajima Y, Come CE, Nardelli P, et al. Luminal plugging on chest CT scan: association with lung function, quality of life, and COPD clinical phenotypes. Chest. 2020;158(1):121-130.
6. Huang BK, Elicker BM, Henry TS, et al. Persistent mucus plugs in proximal airways are consequential for airflow limitation in asthma. JCI Insight. 2024;9(3):e174124.
7. Mettler SK, Nath HP, Grumley S, et al. Silent airway mucus plugs in COPD and clinical implications. Chest. 2024;166(5):1010-1019. Preprint. Posted online November 25, 2023.
8. Mettler SK, Sonavane S, Grumley S, et al. Airway-occluding mucus plugs and cause-specific mortality in COPD. Am J Respir Crit Care Med. 2024;209(12):1508-1510.
9. Nordenmark LH, Hellqvist Å, Emson C, et al. Tezepelumab and mucus plugs in patients with moderate-to-severe asthma. NEJM Evid. 2023;2(10):EVIDoa2300135.
10. Addante A, Raymond W, Gitlin I, et al. A novel thiol-saccharide mucolytic for the treatment of muco-obstructive lung diseases. Eur Respir J. 2023;61(5):2202022.